David Baxter PhD
Late Founder
The neurobiology of fear
Neurophilosophy Blog
October 31, 2007
Fear, that most primitive of emotions, is good, at least when it is kept under control. It is essential for survival, allowing an organism to detect a potential threat to its life. Too much fear, however, can lead to pathological conditions such as anxiety, phobia, paranoia, or post-traumatic stress disorder.
The neural circuitry which processes information about fear is well mapped, but otherwise, little else is known about the biological basis of this emotion. In recent years, however, neuroscientists elucidated some of the cellular and molecular mechnisms underlying fear. A greater understanding of these mechanisms could lead to new treatments for various neuropsychiatric conditions.
Although the word fear is hard to define, everyone knows how it feels to be afraid. The physiological response to fear is called the 'fight or flight' response, or the 'acute stress response', and was first described by the American physiologist Walter B. Cannon in the 1929. The response is caused by the actions of adrenaline, noradrenaline and the steroid cortisol, whose release is triggered by the sympathetic branch of the autonomic nervous system: heart rate increases and breathing speeds up; pupils dilate to let in more light, enabling one to see better; metabolism of fat and glucose in the liver increase to provide the energy that might be needed to escape; production and release of endorphins is stepped-up; and the brain's decision-making centres become primed for action.
Fear can broadly be characterized into two types. Conditioned fear is the process by which an insignificant and otherwise harmless stimulus becomes, by association, a sign of danger. The association between an innocuous stimulus and a fear response can easily be induced by classical conditioning, in which the stimulus is repeatedly associated with something scary, until the stimulus itself is feared. In contrast, unconditioned fear is not learnt, but, rather, is 'instinctive', and can be regarded as an evolutionary relic - we sometimes fear what our ancestors feared.
The amygdala, a small, almond-shaped part of the brain, is known to be involved in emotions, including fear. It is also involved in the recognition of facial expressions and, therefore, of other peoples' emotions. People with damage to the amygdala have difficulty recognizing facial expressions in others. Last year, researchers at the National Institute of Mental health reported that teenagers with bipolar disorder may misread the facial expressions of others; when asked to rate the hostility of a neutral facial expression, the amygdala was shown to be more active these teenagers than in others without the condition. Imaging studies show that, when people are presented with phobia-specific stimuli, the amygdala is activated more rapidly in those people with that particular phobia than in others without it.
The amygdala is part of the limbic system, a complex set of structures that includes the thalamus (or "deep chamber"), the hippocampus, hypothalamus, and olfactory bulb. It is attached to the anterior of hippocampus, and is involved, among other things, in encoding and consolidating memories of fearful experiences. The amygdala receives its main inputs from the visual, auditory and somatosensory cortices, and its main outputs are to the hypothalamus, which controls hormone production and homeostasis, and autonomic centres in the brainstem. The amygdala therefore couples these sensory stimuli (something scary) to the fight-or-flight response.
Most investigators now believe that the association between conditioned and unconditioned stimuli that occurs in fear conditioning involves synaptic plasticity in the amygdala. That is, learning the association between the stimuli requires the strengthening of connections in the lateral nucleus of the amgydala, which consolidates the memory of the association.
Neurons in one part of the lateral amygdala (the dorsal subnucleus) exhibit the sort of properties that would be required for fear conditioning. These cells receive inputs from sensory regions of the thalamus, and also from the cerebral cortex. The former inputs encode conditioned stimuli (e.g. a specific sound), and the latter encode unconditioned stimuli (e.g. an electric shock that becomes associated with that sound).
Because these different inputs converge on the same neurons, the response of the cells is enhanced when both the conditioned and unconditioned stimuli are presented together. This plasticity is short-lived, however. Once the association has been extinguished, and the animal no longer fears the conditioned stimulus, the responses of an adjacent group of cells become enhanced instead. This may explain why fears that have been treated successfully can sometimes come flooding back after a stressful event.
It was known for some time that the encoding and consolidation of fearful memories can be blocked by inhibiting protein synthesis in the amygdala, but it was not clear which proteins are involved. Recently, however, Gleb Shumyatsky and his colleagues at Rutgers University in New Jersey discovered several genes that are highly expressed in the amygdala, and which appear to be involved in this process. One of these encodes a protein called stathmin (also known as oncoprotein 18), which is now known to be involved in mediating the formation of memories of both conditioned and unconditioned fear. There is a high level of expression of the stathmin gene, and a corresponding high concentration of stathmin protein, in the amygdala, but not in the adjacent hippocampus.
Mutant mice lacking the stathmin gene were unable to learn new fears or to act instinctively in a fearful situation, i.e. they had weaker memories of fearful experiences. The stathmin knockout mice also showed less anxiety when presented with new mazes to explore or with potentially dangerous situations. Upon further examination, it was observed that mice lacking the stathmin gene had a less dynamic microtubule network than wild type (normal) mice. Memories are formed by the establishment of new synaptic connections, which require a re-arrangement of microtubules. In the absence of the stathmin protein, microtubules aren't re-arranged so easily, and, as a consequence, the synapses that would normally be modified during memory formation are not as plastic as they should be.
More recently, researchers have used functional magnetic resonance imaging (fMRI) to show that the rostral anterior cingulate cortex (rACC) modulates activity in the amygdala, effectively acting as an 'on-off' switch. Joy Hirsch and her colleagues at Columbia University used a variant of the Stroop test, which involves presenting words for colours, which are printed in a colour that differs from that of the meaning of the word (e.g. green). This discrepancy leads to a delay in the processing of the visual information, increasing the reaction time taken to perform the task.
Instead of using words, Hirsch's team presented participants in their experiments with photographs of happy or scared faces with the word 'HAPPY' or 'FEAR' written across them. It was found that the amygdala was activated before the rACC when the participants were presented with a happy face with the word 'FEAR' printed across it. Soon afterwards, though, the activity in the amygdala would be reduced. That is, initially, the amygdala processes the word 'FEAR', but, soon afterwards, the rACC processes the happy face, and inhibits activity in the amygdala to reduce the fear response. In contrast, when participants were presented with a photograph of a scared face which had the word 'FEAR' written across it, the rACC remained inactive, while activity in the hippocampus persisted for longer.
When we are faced with fearful stimuli but know that we are not in danger, the fight or flight response produced by fear can be enjoyable. Hence, people enjoy taking part in extreme sports such as bungee jumping or sky-diving. This is also why some people enjoy watching horror films. The ability to enjoy fear enables us to try new activities, to explore novel environments, and to be daredevils. Although we have gained some understanding of the neural mechanisms underlying fear and other emotions, this understanding is very basic, and much remains to be discovered. Further insights into how the brain processes fearful information will provide new methods, both cognitive and pharmacological, for treating conditions in which fear has become pathological, such as post-traumatic stress disorder.
References:
Neurophilosophy Blog
October 31, 2007
Fear, that most primitive of emotions, is good, at least when it is kept under control. It is essential for survival, allowing an organism to detect a potential threat to its life. Too much fear, however, can lead to pathological conditions such as anxiety, phobia, paranoia, or post-traumatic stress disorder.
The neural circuitry which processes information about fear is well mapped, but otherwise, little else is known about the biological basis of this emotion. In recent years, however, neuroscientists elucidated some of the cellular and molecular mechnisms underlying fear. A greater understanding of these mechanisms could lead to new treatments for various neuropsychiatric conditions.
Although the word fear is hard to define, everyone knows how it feels to be afraid. The physiological response to fear is called the 'fight or flight' response, or the 'acute stress response', and was first described by the American physiologist Walter B. Cannon in the 1929. The response is caused by the actions of adrenaline, noradrenaline and the steroid cortisol, whose release is triggered by the sympathetic branch of the autonomic nervous system: heart rate increases and breathing speeds up; pupils dilate to let in more light, enabling one to see better; metabolism of fat and glucose in the liver increase to provide the energy that might be needed to escape; production and release of endorphins is stepped-up; and the brain's decision-making centres become primed for action.
Fear can broadly be characterized into two types. Conditioned fear is the process by which an insignificant and otherwise harmless stimulus becomes, by association, a sign of danger. The association between an innocuous stimulus and a fear response can easily be induced by classical conditioning, in which the stimulus is repeatedly associated with something scary, until the stimulus itself is feared. In contrast, unconditioned fear is not learnt, but, rather, is 'instinctive', and can be regarded as an evolutionary relic - we sometimes fear what our ancestors feared.
The amygdala, a small, almond-shaped part of the brain, is known to be involved in emotions, including fear. It is also involved in the recognition of facial expressions and, therefore, of other peoples' emotions. People with damage to the amygdala have difficulty recognizing facial expressions in others. Last year, researchers at the National Institute of Mental health reported that teenagers with bipolar disorder may misread the facial expressions of others; when asked to rate the hostility of a neutral facial expression, the amygdala was shown to be more active these teenagers than in others without the condition. Imaging studies show that, when people are presented with phobia-specific stimuli, the amygdala is activated more rapidly in those people with that particular phobia than in others without it.
The amygdala is part of the limbic system, a complex set of structures that includes the thalamus (or "deep chamber"), the hippocampus, hypothalamus, and olfactory bulb. It is attached to the anterior of hippocampus, and is involved, among other things, in encoding and consolidating memories of fearful experiences. The amygdala receives its main inputs from the visual, auditory and somatosensory cortices, and its main outputs are to the hypothalamus, which controls hormone production and homeostasis, and autonomic centres in the brainstem. The amygdala therefore couples these sensory stimuli (something scary) to the fight-or-flight response.
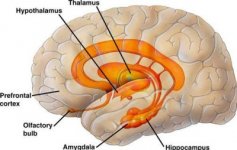
Most investigators now believe that the association between conditioned and unconditioned stimuli that occurs in fear conditioning involves synaptic plasticity in the amygdala. That is, learning the association between the stimuli requires the strengthening of connections in the lateral nucleus of the amgydala, which consolidates the memory of the association.
Neurons in one part of the lateral amygdala (the dorsal subnucleus) exhibit the sort of properties that would be required for fear conditioning. These cells receive inputs from sensory regions of the thalamus, and also from the cerebral cortex. The former inputs encode conditioned stimuli (e.g. a specific sound), and the latter encode unconditioned stimuli (e.g. an electric shock that becomes associated with that sound).
Because these different inputs converge on the same neurons, the response of the cells is enhanced when both the conditioned and unconditioned stimuli are presented together. This plasticity is short-lived, however. Once the association has been extinguished, and the animal no longer fears the conditioned stimulus, the responses of an adjacent group of cells become enhanced instead. This may explain why fears that have been treated successfully can sometimes come flooding back after a stressful event.
It was known for some time that the encoding and consolidation of fearful memories can be blocked by inhibiting protein synthesis in the amygdala, but it was not clear which proteins are involved. Recently, however, Gleb Shumyatsky and his colleagues at Rutgers University in New Jersey discovered several genes that are highly expressed in the amygdala, and which appear to be involved in this process. One of these encodes a protein called stathmin (also known as oncoprotein 18), which is now known to be involved in mediating the formation of memories of both conditioned and unconditioned fear. There is a high level of expression of the stathmin gene, and a corresponding high concentration of stathmin protein, in the amygdala, but not in the adjacent hippocampus.
Mutant mice lacking the stathmin gene were unable to learn new fears or to act instinctively in a fearful situation, i.e. they had weaker memories of fearful experiences. The stathmin knockout mice also showed less anxiety when presented with new mazes to explore or with potentially dangerous situations. Upon further examination, it was observed that mice lacking the stathmin gene had a less dynamic microtubule network than wild type (normal) mice. Memories are formed by the establishment of new synaptic connections, which require a re-arrangement of microtubules. In the absence of the stathmin protein, microtubules aren't re-arranged so easily, and, as a consequence, the synapses that would normally be modified during memory formation are not as plastic as they should be.
More recently, researchers have used functional magnetic resonance imaging (fMRI) to show that the rostral anterior cingulate cortex (rACC) modulates activity in the amygdala, effectively acting as an 'on-off' switch. Joy Hirsch and her colleagues at Columbia University used a variant of the Stroop test, which involves presenting words for colours, which are printed in a colour that differs from that of the meaning of the word (e.g. green). This discrepancy leads to a delay in the processing of the visual information, increasing the reaction time taken to perform the task.
Instead of using words, Hirsch's team presented participants in their experiments with photographs of happy or scared faces with the word 'HAPPY' or 'FEAR' written across them. It was found that the amygdala was activated before the rACC when the participants were presented with a happy face with the word 'FEAR' printed across it. Soon afterwards, though, the activity in the amygdala would be reduced. That is, initially, the amygdala processes the word 'FEAR', but, soon afterwards, the rACC processes the happy face, and inhibits activity in the amygdala to reduce the fear response. In contrast, when participants were presented with a photograph of a scared face which had the word 'FEAR' written across it, the rACC remained inactive, while activity in the hippocampus persisted for longer.
When we are faced with fearful stimuli but know that we are not in danger, the fight or flight response produced by fear can be enjoyable. Hence, people enjoy taking part in extreme sports such as bungee jumping or sky-diving. This is also why some people enjoy watching horror films. The ability to enjoy fear enables us to try new activities, to explore novel environments, and to be daredevils. Although we have gained some understanding of the neural mechanisms underlying fear and other emotions, this understanding is very basic, and much remains to be discovered. Further insights into how the brain processes fearful information will provide new methods, both cognitive and pharmacological, for treating conditions in which fear has become pathological, such as post-traumatic stress disorder.
References:
- Larson, C. L., et al. (2006). Fear is fast in phobic individuals: Amygdala activation in response to fear-relevant stimuli. Biol. Psychiatry 60: 410-417. [Full text]
- Nader, K. et al. (2000). Fear memories require protein synthesis in the amygdala for reconsolidation after retrieval. Nature 406: 722-726. [Full text]